Episode 16: Multicellularity in cyanobacteria
One of the most significant events in Earth’s history has been the oxygenation of its atmosphere 2.45–2.32 billion years ago. This accumulation of molecular oxygen in Earth’s atmosphere was so significant that it is now commonly known as the Great Oxidation Event (GOE). The long-reaching effects of the GOE were literally world-changing; the compositions of the atmosphere and hydrosphere were altered, and through various redox reactions (where atoms have their oxidation state changed), the nature of the continents and global climate changed too. However, and perhaps from an anthropocentric viewpoint, the most important effect would be upon the biosphere: the GOE paved the way for the evolution of aerobic (oxygen respiring) organisms, including our earliest ancestors.
It is possible to track the GOE through geochemical traces left in the rock record. However, one thing we’re still uncertain about is whether or not this event represents either a sharp increase in oxygen production or a reduction in oxygen sinks – the jury is still out on that one. With this level of ambiguity over the dynamics of the GOE, it may be a little surprising to know that there has been a long-standing consensus on how the oxygen was actually produced: photosynthetic organisms called cyanobacteria (blue-green algae, so named after the pigment they produce).
In this episode we discuss with Dr. Bettina Schirrmeister (University of Bristol) about the evolution of cyanobacteria and the role they played in the GOE.
Podcast: Download (Duration: 23:16 — 31.9MB)
Cyanobacteria represent one of the most morphologically diverse groups of prokaryotic organisms (Bacteria and Archaea). They are classically separated into their various biological groups by their morphology; some groups are unicellular, others are multicellular and then there are those that can produce differentiation between cells. However, genetic studies show that this morphological grouping is not representative of actual biological relationships between cyanobacteria and that morphological characteristics such as multicellularity evolved independently in several lineages.

The morphologies of cyanobacteria (unicellular and multicellular taxa). Classically cyanobacteria have been grouped into five ‘sections’ according to their morphology, with sections I and II (not pictured) describing unicellular taxa and section III–V comprising multicellular species.
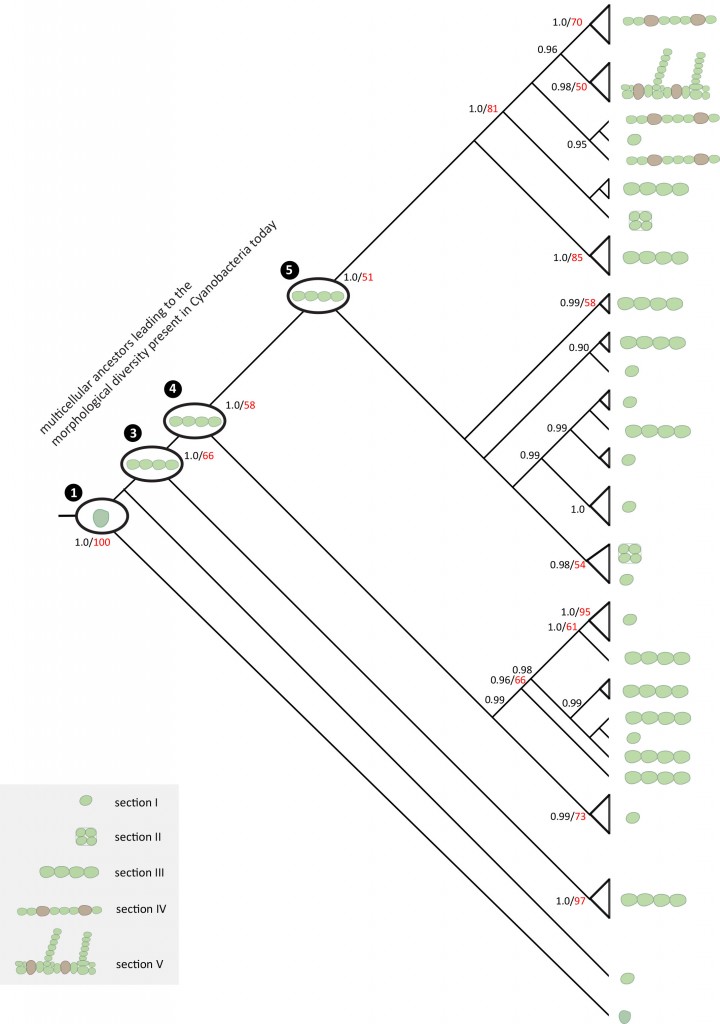
Molecular data have shown that, in fact, none of the five morphologies are representative of true biological diversity. In other words, multicellularity has evolved independently in many different groups.
Fortunately, relative to other prokaryotes, cyanobacteria have a well-studied fossil record. This has led to the realisation that much of the diversity in modern forms existed early on in the evolutionary history of the group; they have in fact changed very little in basic form over billions of years. This is best exemplified in the 2 billion year old Gunflint Chert, where the first unequivocal evidence of cyanobacteria can be found. Single-celled (coccoidal) and multicellular (filamentous) forms are both present and show very little superficial variation from their modern counterparts.
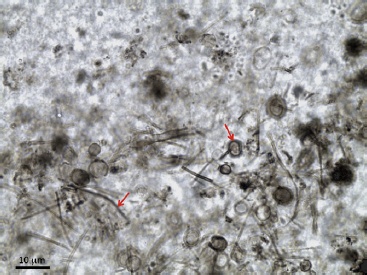
Thin section photomicrograph showing multiple examples of spheroidal (Huroniospora) and filamentous (Siphonophycus plus possible Gunflintia) microfossils from a stromatolitic chert within the ∼1900 million year old Gunflint Formation, Canada. From Wacey et al. (2012) Precambrian Research 220-221: 234-250.
Prior to this, potential evidence for cyanobacterial existence is found in the form of trace-fossils, known as stromatolites. These are large sedimentary structures, formed by the interaction of photosynthetic bacterial mats (commonly, but not always, cyanobacteria) and sediment. The bacterial mats catch and encrust sediments around them, forming a consolidated calcareous structure. The photosynthetic bacteria then migrate through to the top surface and the cycle repeats eventually forming large, layered columns. Stromatolites have been observed as long as around 3.45 billion years ago, persisting through to the modern day, suggesting that photosynthetic organisms were present at this time, even if not necessarily cyanobacteria.
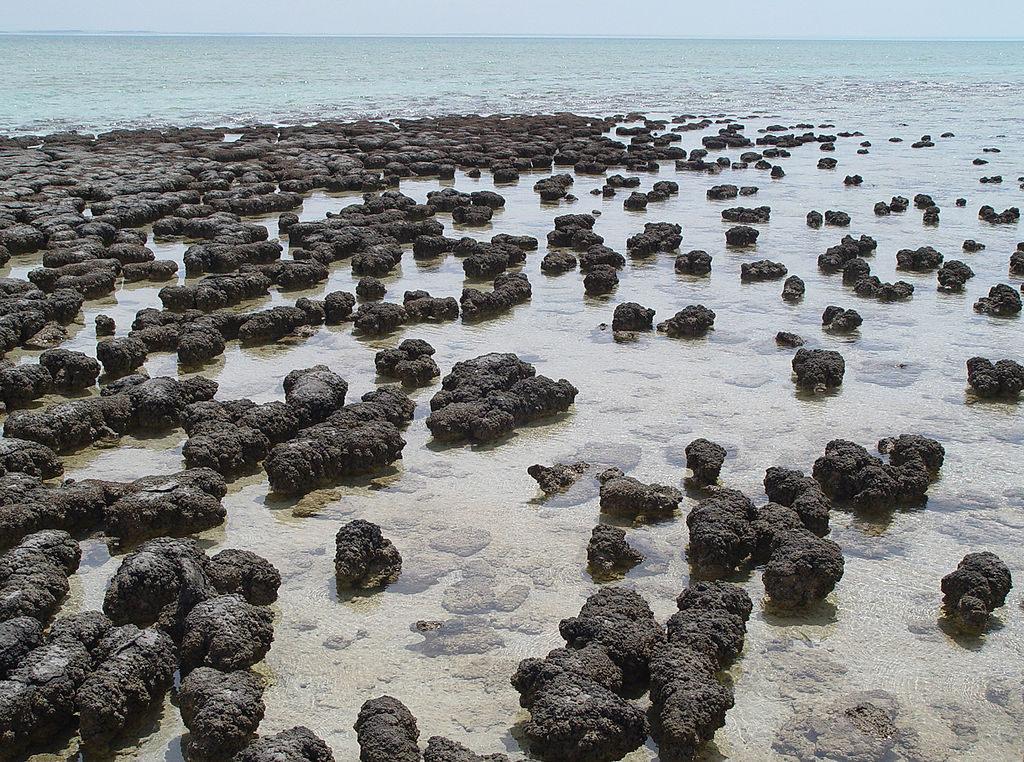
Modern stromatolites growing in Hamelin Pool Marine Nature Reserve, Shark Bay in Western Australia. Image credit: Paul Harrison CC BY-SA 3.0.
Although cyanobacteria are fairly well accepted as the main source of oxygen for the GOE, their precise role in the event remains unclear. Were they indirect contributors, having existed fairly unchanged for billions of years, when at 2.45 billion years ago tectonic events caused the cessation of oxygen sinks? Or were they the driving force behind the GOE representing a biological revolution in cyanobacterial forms? Without discovery of any direct fossil evidence older than the 2 billion year old Gunflint Chert this may seem an impossible question to ask, yet a team from the University of Zurich, led by Dr. Bettina Schirrmeister, conducted a phylogenetic analysis of living and fossil cyanobacteria in the hope of providing an answer.
Phylogenetic analyses are statistical methods for describing the relationships between any group of individuals based on their shared characteristics. This provides not only a quantifiable hypothesis of relationships between organisms, but a hypothetical evolutionary ‘pathway of least resistance’ through which each organism evolved, depending on the precise reconstruction method applied. Even though a hypothetical family tree, including all of its relationships and evolutionary branches, is produced, unless each branch has a temporal association, it is useless in answering the questions as to the relation of cyanobacteria to the GOE. This is where the fossil record of the cyanobacteria can be used. If you are able to assign a fossil to a higher taxonomic group, then you can assign a minimum age of origin for that group. However, bearing in mind that the fossil record of cyanobacteria only exists from around 2 billion years ago, how could they estimate times of evolution for those groups beyond this point?
This is where genetic studies of extant cyanobacteria come into play. By determining the differences between cyanobacterial genomes and dividing by observed mutation rates in modern specimens, divergence times can be estimated and incorporated into our temporally-constrained evolutionary tree. The study showed that all extant lineages of cyanobacteria existed from before the GOE in the Archaean Eon; a result that was repeated in all reruns and variations of the analysis. This was not particularly surprising given the diversity of fossils already present in the Gunflint Chert and the rarity of fossil yielding localities. However there were some significant coincidences between the GOE and hypothesised cyanobacterial divergence events. The evolution of multicellularity was resolved at, or just before, the onset of the GOE, with the emergence of the majority of the modern lineages shortly after the accumulation of atmospheric oxygen.
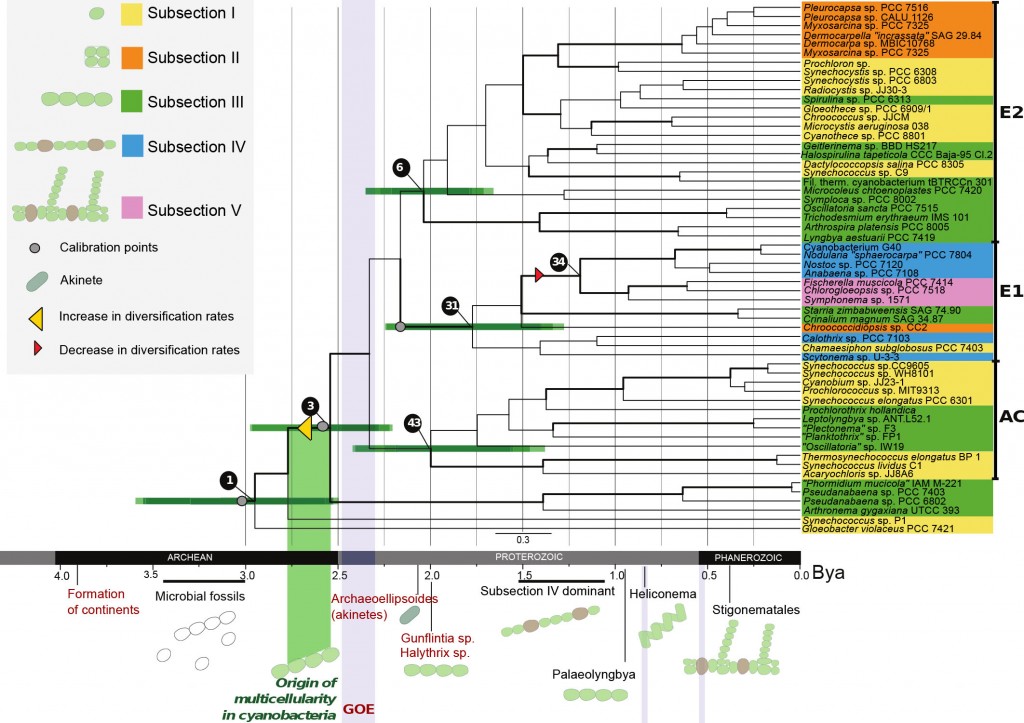
By applying phylogenetic methods on living taxa and combining results with fossil estimates the origin of multicellularity in cyanobacteria could be estimated. The results support theories which suggest an origin of cyanobacteria in the Archean Eon and an evolution of multicellularity before the Great Oxidation Event.
What this shows is that the cause of the GOE, at least in part, was not the ascendance of any particular group of cyanobacteria, but a revolution of form in multiple lineages. Multicellularity would have enabled an increase in motility and produced favourable metabolic economies of scale, both obvious advantages. As a respiratory by-product, oxygen would most likely have been toxic to much of the biota. Increased oxygen levels would likely have created an evolutionary pressure on the cyanobacteria, and in response, driving their evolutionary radiation into the diversity of forms we see today.
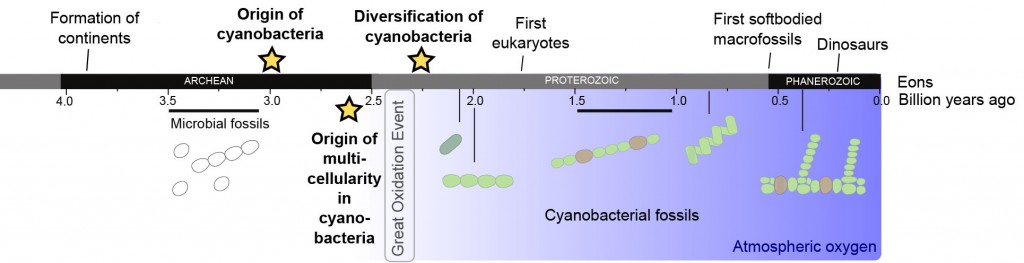
Timeline of the evolution of Earth. Continents formed ~3.8 Bya, after temperatures had cooled and meteorite bombardment had stopped. In ~3.46 billion year old rocks (Australia) first evidence for life is found. Around 2.45–2.32 Bya oxygen accumulated in the atmosphere during the Great Oxidation Event produced by cyanobacterial oxygenic photosynthesis. Oldest cyanobacterial fossils can be found in the Gunflint Chert (1.88-2.0 Bya). First eukaryotes evolve around 1.6-1.8 Bya.
However, in identifying this concurrence, many more questions regarding the evolution of multicellularity are raised: why did it occur when it did? Was it the sole driving force behind the GOE? The one thing we do know is that this association exists and it paved the way for the evolution of the planet as we now know it.
Unless otherwise stated, all images from Schirrmeister, B. E. et al.: Evolution of multicellularity coincided with increased diversification of cyanobacteria and the Great Oxidation Event, PNAS, 110(5), 1791–1796, 2013.